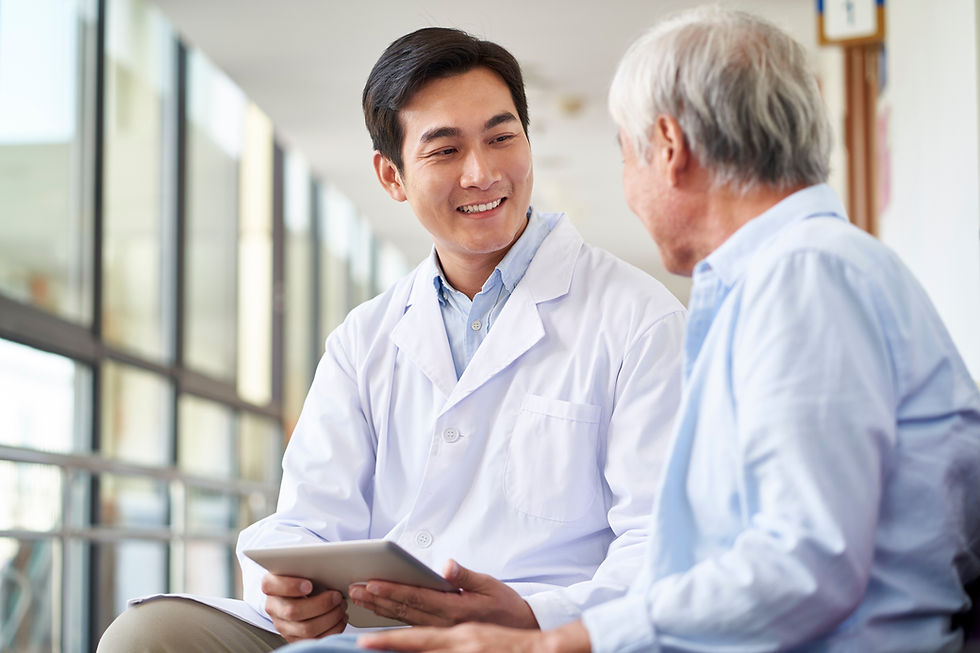
Historical Context
Since its initial identification by James Parkinson in 1817, Parkinson's disease has remained a significant focus in the field of neurology. In his seminal paper, "The Shaking Palsy," Parkinson meticulously recorded the symptoms of the condition, then referred to as Paralysis agitans. In wonderfully succinct and pithy English, Parkinson captured the clinical picture:
“Involuntary tremulous motion, with lessened muscular power, in parts not in action and even when supported; with a propensity to bend the trunk forward, and to pass from a walking to a running pace: the senses and intellects being uninjured.”
More than 50 years later, Jean-Martin Charcot at the Salpêtrière hospital in Paris demonstrated a more comprehensive understanding of Parkinson's disease, distinguishing bradykinesia as a defining feature of the illness:
“Even before rigidity manifests, patients already struggle with everyday tasks, which can be attributed to another cause. In some of the cases I have presented, you can easily observe their difficulty in executing movements, even when rigidity or tremor is not the predominant factor. A simple examination reveals that their challenge lies more in the slowness of movement rather than actual weakness. Despite experiencing tremors, these patients can still perform most tasks, but they do so with remarkable slowness. There is a noticeable delay between their thoughts and actions, suggesting that neural activity requires significant effort.”
Charcot and his students extensively described the clinical spectrum of Parkinson's disease, identifying two main prototypes: the tremorous and the rigid/akinetic forms. They provided detailed insights into the arthritic changes, dysautonomia, and pain that can accompany Parkinson's. Charcot was the first to name the diseases as "Parkinson's Disease" in honour of Dr. Parkinson's pioneering work.
Most prevalent neurodegenerative disorder 2024
Today, Parkinson's stands as one of most prevalent neurodegenerative disorders, affecting over 6 million individuals worldwide with around 145,000 patients in the UK. This figure reflects a remarkable 2.5-fold increase compared to previous generations, and disconcertingly, the incidence is projected to double in the next generation. As practitioners and interested readers, it is crucial to grasp the complexities of this condition, its evolving prevalence, and the pressing need for advancements in research, treatment, and holistic interventions to improve patient care and outcomes.
“I am not so much a Parkinson’s sufferer, as I am someone learning to live well with Parkinson’s.”
JT, London
Dopamine 101:
A quick overview of how dopamine is made:
Dopamine metabolism is a complex process that involves the conversion of dietary protein to enable the synthesis of neurotransmitters, including dopamine and adrenaline. Here's a simplified overview of the steps involved:
1. Dietary Protein: Dopamine metabolism starts with the consumption of dietary protein from food. Proteins are broken down into amino acids during digestion.
2. Tyrosine: One of the essential amino acids derived from dietary protein is tyrosine. Tyrosine is a precursor for the synthesis of dopamine.
3. Tyrosine Hydroxylase: In the brain, tyrosine is acted upon by an enzyme called tyrosine hydroxylase, which converts tyrosine into L-dihydroxyphenylalanine (L-DOPA).
4. L-DOPA: L-DOPA is a key intermediate in dopamine synthesis. It crosses the blood-brain barrier and enters the brain, where it serves as a precursor for dopamine.
5. Dopamine: Inside the brain, L-DOPA is further converted into dopamine by the enzyme aromatic L-amino acid decarboxylase (AADC).
6. Dopamine Transporter: Once synthesised, dopamine is stored in vesicles within nerve terminals until it is needed for neurotransmission. When a nerve signal is sent, dopamine is released into the synapse.
7. Receptors: Dopamine binds to specific receptors on the postsynaptic neuron, transmitting the nerve signal and producing various physiological and behavioural effects.
8. Dopamine Reuptake: After its action, dopamine is rapidly taken back into the presynaptic neuron through a process known as reuptake, mediated by the dopamine transporter (DAT).
9. Dopamine can be further metabolised into norepinephrine (noradrenaline), which in turn can be converted into adrenaline (epinephrine) through the action of phenylethanolamine N-methyltransferase (PNMT) in the adrenal medulla.
In summary, dopamine metabolism begins with the breakdown of dietary protein into amino acids, including tyrosine. Tyrosine is then converted to L-DOPA, which serves as the precursor for dopamine synthesis. Once synthesised, dopamine is involved in neurotransmission, and it can also be further metabolised into norepinephrine and adrenaline. These neurotransmitters play essential roles in various physiological processes, including motor control, mood regulation, and stress response.
Pathophysiology of Parkinson's: Understanding the Neurological Progression
Parkinson's disease (PD) is a progressive neurodegenerative disorder that primarily targets the brain's motor system, leading to a range of physical and potential cognitive impairments. The pathophysiology of PD is complex and involves multiple factors contributing to its development and progression.
The main pathological feature of PD is the loss of dopamine-producing neurons in a region of the brain called the substantia nigra, which is responsible for producing dopamine, a neurotransmitter critical for coordinating movement and regulating various brain functions. As these neurons degenerate, dopamine levels decrease, leading to disturbances in motor control.
The primary cause, or causes, of PD remain largely unknown, but both genetic and environmental factors are thought to play significant roles. Around 10-15% of cases are considered familial, linked to specific genetic mutations. However, the vast majority of cases are sporadic, arising from a combination of genetic susceptibility, nutritional and lifestyle factors, and exposure to environmental toxins.
One key protein implicated in PD is alpha-synuclein. In healthy neurons, alpha-synuclein aids in maintaining synaptic vesicles responsible for neurotransmitter release. However, in PD, alpha-synuclein aggregates abnormally, forming clumps known as Lewy bodies. The Lewy bodies disrupt cellular function and contribute to neuronal death.
The loss of dopamine leads to an imbalance between dopamine and other neurotransmitters, particularly acetylcholine. This imbalance causes a dysregulation of the basal ganglia circuitry, a group of interconnected brain structures involved in motor control. This disruption results in the characteristic motor symptoms of PD, such as resting tremors, bradykinesia (slowness of movement), rigidity, and postural instability.
As PD progresses, other brain regions beyond the substantia nigra are also affected, leading to non-motor symptoms. These may include cognitive impairments, mood disorders, sleep disturbances, and autonomic dysfunction.
Recent research suggests that the pathological process in PD begins years before clinical symptoms become apparent. By the time motor symptoms manifest, a significant amount (estimated at 80%) of dopaminergic neurons are already thought to be lost. This emphasises the need for early detection and a holistic, person-centred intervention.
Differential Diagnosis for Parkinson's
Atypical Parkinsonism:
While PD is the primary cause of Parkinsonism, similar symptoms can also arise from Atypical Parkinsonism (AP). AP may result from other underlying disorders, prescription medications (particularly those used in the treatment of psychiatric conditions), or exposure to toxic substances such as mercury or carbon monoxide. Several neurodegenerative disorders can lead to Atypical Parkinsonism. Examples include multiple system atrophy (MSA) and corticobasal degeneration (CBD). Each of these disorders presents unique patterns of brain degeneration and clinical features that differentiate them from typical PD.
Another important differential diagnosis is progressive supranuclear palsy (PSP). PSP is a rare and progressive neurodegenerative disorder that affects the brain's control of movement and balance. It is caused by the abnormal accumulation of tau protein in brain cells. Tau is a vital protein involved in maintaining the structure and stability of neurons. However, in PSP, there is a malfunction in the way tau is processed and regulated, leading to the formation of toxic aggregates or "tau tangles" within the brain. These tangles disrupt the normal functioning of neurons, particularly those in the basal ganglia and brainstem, which are responsible for coordinating voluntary movements. PSP presents with early and prominent balance problems and falls, followed by difficulties in eye movements, particularly looking down and up. Unlike PD, tremors are less common in PSP, and response to levodopa, a primary PD medication, is generally limited in PSP patients. Cognitive changes, including executive function impairment, are more pronounced in PSP, distinguishing it further from PD.
Parkinson’s Disease Symptoms
The initial signs of PD can be challenging to differentiate from normal ageing, but common symptoms include tremors, stiffness, and difficulties with walking. Not all symptoms manifest in every individual, but additional symptoms do often develop as the disease progresses.
Motor Symptoms:
The classic symptom of PD is a slow tremor in the hand while at rest, disappearing when the hand is voluntarily moved. As the disease advances, tremors may affect both hands, arms, legs, head, or jaw. Tremors are more noticeable during relaxation and reduce during movement or sleep.
Bradykinesia, or slowness of movement, is a cardinal symptom found in all cases of PD. It involves difficulty initiating movement (akinesia) and the movement taking longer to perform. As the disease progresses, body stiffness affects both sides, making everyday functions challenging. Walking becomes progressively more difficult and slow, often leading to shuffling or falls due to stiffness and loss of autonomic control.
Other motor symptoms include oral motor disorders and dystonia (sustained muscular contraction with abnormal movements), and postural deformities characterised by abnormally flexed body posture. Early intervention by a speech and language therapist is essential to help maintain the integrity and power of the voice.
Neuropsychiatric Symptoms:
Cognitive symptoms may occur early in the disease and become more prevalent as it progresses. These may include impairment in language, memory, visual-spatial skills, and executive functions, such as planning and abstract thinking. Neuropsychiatric symptoms can also include sleep disorders, mood symptoms like apathy, depression, and anxiety, as well as impulse control disorders, psychosis, and cognitive impairment in later stages.
In the later stages of PD, PD dementia is commonly experienced, leading to additional deficits in recognition memory, attention processes, and visual perception. 1
.
In addition to the motor and neuropsychiatric symptoms mentioned earlier, PD can give rise to a range of other symptoms that significantly impact a patient's quality of life. These non-motor symptoms can often be overlooked or attributed to other causes, making their recognition and management essential in the comprehensive care of individuals with PD .
Orthostatic Hypotension:
PD may lead to a drop in blood pressure upon standing, causing dizziness or fainting. Orthostatic hypotension can contribute to falls and the trauma of a fall leads to further complications.
Gastrointestinal Issues:
Slow-transit constipation is a common problem in PD, resulting from reduced bowel motility. This condition can cause discomfort and affect the patient's overall wellbeing. Nutritional and dietary interventions often prove to be the best options. Magnesium oxide has a proven use as an aid to regular bowel motions in PD. 2
Urinary Control Disturbances:
PD can lead to urinary frequency, urgency, incontinence, and nocturia, impacting the patient's daily routine and sleep patterns. Falls are also more common at night when the PD patient may need to use the toilet.
Erectile Dysfunction in Males:
Male patients with PD may experience difficulties with sexual function due to changes in nervous system control.
Sleep Problems:
Sleep disturbances are prevalent in PD, characterised by shallow sleep, frequent waking, and difficulties in falling or staying asleep. These issues can contribute to daytime fatigue and worsen other symptoms. 3
Reduced or Lost Sense of Smell and Taste:
Olfactory dysfunction is common in PD, affecting the patient's ability to smell and taste, potentially leading to reduced appetite and weight loss.
Musculoskeletal Pain:
Patients with PD often experience musculoskeletal pain, which can be due to altered posture, rigidity, and abnormal movements. This pain can affect various body parts and interfere with daily activities.
Recognising and addressing these non-motor symptoms is essential in the overall management of PD. Proper evaluation, appropriate therapies, and lifestyle modifications can help alleviate these symptoms, improving the patient's overall comfort and well-being. A holistic approach to care, which considers both motor and non-motor symptoms, can lead to a better quality of life for individuals living with PD, their families, and caregivers.
Incidence of Parkinson's Disease:
Age-related Patterns and Gender Disparities
PD typically manifests its symptoms after the age of 50, with an average onset around 60 years. However, later onset or diagnosis is not uncommon. In rarer cases, young or early onset Parkinson's Disease (YOPD) occurs in individuals diagnosed before the age of 40, while juvenile PD, diagnosed before the age of 18, is exceptionally rare.
This age-related neurodegenerative disorder affects approximately 3% of the population by the age of 65, with the prevalence increasing to about 5% among individuals over 85 years old. As life expectancy rises, the incidence of PD is becoming more pronounced in the elderly population.
PD displays a gender disparity, affecting men twice as often as women.4 Women, however, experience a higher mortality rate and a faster progression of the disease compared to their male counterparts. Some holistic PD specialists are looking at the link between menopause and the potential decline in both cognitive and neurological function. A full hormonal analysis with a view to supplementing with bio-identical hormones may well play a contributing role in both prevention and the management of PD in women.
As research and awareness continues to grow, understanding the age-related patterns and gender differences in PD incidence becomes crucial for accurate diagnosis, effective management, and the development of targeted interventions to improve the quality of life for those affected by this complex neurological disorder.
Diagnosis
Diagnosis is most often based on clinical observation of symptoms and the systematic ruling out other potential causes or conditions through tests. Healthcare professionals should suspect PD in individuals presenting with tremor, stiffness, slowness, balance, or gait problems. Referral to a specialist in differential diagnosis of PD is recommended before initiating treatment, and diagnosis should follow the UK Parkinson's Disease Society Brain Bank Criteria, a set of diagnostic guidelines used to classify and confirm PD neuropathologically. Regular reviews are essential to monitor progression.
PD is also diagnosed by DAT scan (Dopamine Transporter Scan), a nuclear medicine imaging technique that assesses dopamine transporter levels in the brain. Reduced uptake of the radiotracer indicates dopamine deficiency, helping to differentiate PD from other conditions with similar symptoms.
Aetiology of Parkinson's
It is widely agreed that, as with most chronic conditions, PD is a multi-factorial condition with several factors exacerbating, contributing to, or leading to the onset. Toxic exposure, genetic predisposition, lifestyle, and diet all play a part.
In this next section we look at some of the well-established causes and explore some of the less known drivers.
Oxidative Stress: 5-9
The cell death within the substantia nigra has been attributed to oxidative stress, inflammation and mitochondrial dysfunction. These drivers are consistent with most chronic disease onset and can be modified by environment, diet and lifestyle.
Oxidative stress arises from the dysregulation of cellular redox activity, where the production of reactive oxygen species (ROS) outweighs clearance by endogenous antioxidant enzymes. The accumulation of ROS mediates neuronal damage. ROS accumulation can result in oxidative damage to lipids, proteins, DNA, and RNA – all of which compromises neuronal function and structural integrity. Increased oxidative stress has been observed in early stages of the disease, prior to significant neuron loss, which implies uncontrolled ROS generation as a causal factor in dopaminergic neuron death, rather than being a secondary response in neurodegeneration.
Glutathione:10-13
One of the first biochemical changes noted in PD is the depletion of glutathione (a major intracellular antioxidant and redox regulator) within the substantia nigra, further demonstrating that oxidative stress is a significant factor in the onset and progression of the condition. Oxidative stress can cause damage to cells and lead to inflammation and apoptosis; this can also lead to mitochondrial dysfunction which will affect the cell’s ability to produce energy and therefore the function of the cell will be significantly reduced. Glutathione depletion has been linked to the aggregation of defective proteins leading to death of dopaminergic neurons and increased susceptibility to endogenous and exogenous toxins.
Neuroinflammation: 14
Chronic neuroinflammation is one of the hallmarks of PD. Microglia is one of the major cell types which are involved in the inflammatory responses in the central nervous system, and the chronic release of pro-inflammatory cytokines by activated astrocytes and microglia leads to dopaminergic neuron degeneration in the substantia nigra. Further biochemical analysis reveals higher levels of pro-inflammatory mediators including tumour necrosis factor-α (TNF-α), interleukin-1β (IL-1β) and interferon-gamma (IFN-γ) in the midbrain of PD patients, which strongly suggests the involvement of immune components in PD’s pathogenesis.
Taken together, oxidative stress and neuroinflammation play a synergistic role in inducing and aggravating PD, and as such, any therapeutic interventions should consider both aspects.
Inflammation and immune dysfunction: 15
Multiple investigations involving the analysis of peripheral blood and cerebrospinal fluid from individuals with PD indicate changes in markers related to inflammation and variations in immune cell populations. These alterations could potentially trigger or worsen neuroinflammation, contributing to the ongoing neurodegenerative process. Within the realm of PD, specific disease-related genes and risk factors have been pinpointed as regulators of immune activity. Moreover, mounting evidence highlights the potential impact of viral or bacterial exposures, pesticide exposure, and disruptions in gut microbiota on the development of the disease. This accumulating knowledge has given rise to the theory that intricate interactions between genes and environmental factors converge with the aging immune system, creating a synergistic scenario known as the 'perfect storm.' This convergence paves the way for the initiation and progression of PD.
The interplay between immune functionality and inflammation frequently receives attention in complementary medicine. This opens up the possibility of incorporating nutritional medicine into strategies for managing PD.
Genetics: 16,17
Genetic factors play a significant role in the development of PD, contributing to both its familial and sporadic forms. While most PD cases are considered sporadic, emerging research has shed light on several genes associated with the inherited form of the disease. Understanding the genetic underpinnings of PD is crucial for unravelling its pathogenesis, developing targeted and combination therapies, and identifying potential risk factors.
Familial PD accounts for around 10% - 15% of all cases and exhibits an autosomal dominant pattern of inheritance, which means that a single copy of the mutated gene from one parent is sufficient to cause the disease. Mutations in genes such as SNCA, LRRK2, PARK2, PINK1, and DJ-1 have been linked to familial PD.
The alpha-synuclein gene (SNCA) was one of the first to be implicated in PD. Mutations or gene duplications of SNCA lead to an overproduction or aggregation of alpha-synuclein protein, contributing to the formation of Lewy bodies, a hallmark pathological feature of PD.
Leucine-rich repeat kinase 2 (LRRK2) gene mutations are the most common genetic cause of familial PD, particularly in certain ethnic groups. LRRK2 encodes a kinase enzyme involved in various cellular processes, and certain mutations result in increased kinase activity, leading to neuronal damage. Parkin (PARK2) and PTEN-induced putative kinase 1 (PINK1) genes are associated with autosomal recessive forms of familial PD. Loss-of-function mutations in these genes impair mitochondrial quality control and cellular homeostasis, leading to neuronal dysfunction and degeneration. DJ-1 (PARK7) mutations are also linked to autosomal recessive PD. The DJ-1 protein plays a role in cellular defence against oxidative stress, and its dysfunction results in increased vulnerability to cellular damage.
Besides familial cases, genome-wide association studies (GWAS) have identified several risk loci associated with sporadic PD. These genetic risk factors include SNCA, LRRK2, GBA, and other genes involved in cellular processes such as autophagy, lysosomal function, and immune response. While these variants may not directly cause PD, they increase susceptibility to the disease, likely through interacting with environmental factors. It is essential to recognise that while genetic factors contribute significantly to PD risk, they do not act alone. PD is considered a complex disease with both genetic and environmental influences. Environmental factors, such as exposure to toxins, pesticides, and heavy metals, may interact with genetic predisposition, leading to the manifestation of the disease in susceptible individuals.
The interplay between genetic and environmental factors in PD pathogenesis remains an area of active research. Gene-environment interactions are believed to be crucial in determining an individual's risk and the progression of the disease. Understanding these complex interactions will pave the way for personalised medicine approaches and potentially new preventive strategies. Advancements in genetic research have also opened avenues for potential disease-modifying therapies. For instance, the identification of LRRK2 mutations in PD has spurred efforts to develop LRRK2-specific inhibitors as a therapeutic approach. Targeting specific genetic pathways involved in PD pathophysiology holds promise for halting or slowing disease progression. Moreover, genetic factors play a role in predicting disease course and response to treatment. For example, certain genetic variants may influence the efficacy of dopamine replacement therapy or deep brain stimulation, guiding clinicians in tailoring treatment strategies to individual patients.
Genetic factors play a crucial role in PD, both in its familial and sporadic forms. Familial cases, albeit relatively rare, have provided key insights into the disease's molecular mechanisms. Additionally, genome-wide association studies have identified risk loci that offer valuable clues to the genetic architecture of sporadic PD. Understanding the genetic underpinnings of Parkinson's is essential for advancing our knowledge of the disease, developing targeted therapies, and potentially identifying modifiable risk factors for disease prevention. As research continues, the integration of genetic information into clinical practice may pave the way for more personalised and effective management of PD.
Mitochondrial Dysfunction: 18,19
Known as the powerhouse of the cell due to their role in generating energy in the form of adenosine 5’ triphosphate (ATP), mitochondria are highly dynamic and have a very complex structure. Mitochondria play a key role in the function and survival of neurons in the brain and defects in mitochondrial respiration are involved in the development and progression of PD. Mitochondrial dysfunction in the dopaminergic neurons of idiopathic and familial PD is well known, although the underlying mechanisms are not clear. Mitochondrial dysfunction is mainly characterized by the generation of ROS, a decrease in mitochondrial complex I enzyme activity, cytochrome-c release, ATP depletion and caspase 3 activation.
Environmental toxins: 20,21
The impact of environmental pollutants on the human population is acknowledged as a substantial influencer in the emergence of PD and related parkinsonism conditions. Although pesticides have consistently emerged as identifiable risk elements for PD, they constitute merely a portion of the array of environmental toxins to which we are routinely exposed. Consequently, substances beyond pesticides, including metals, solvents, and other organohalogen compounds have also been linked to the observable clinical and pathological characteristics of these movement-related disorders.
Recent research has revealed a compelling link between PD and exposure to environmental toxins, encompassing the following substances:
· Pesticides: Various pesticides, including organochlorines, glyphosate, carbamates, paraquat, maneb, rotenone, pyrethroid, and diethyldithiocarbamate, have been associated with an increased risk of PD 22,23.
· Trace Metals: Certain trace metals such as copper, iron, and manganese have been implicated in PD. These elements, even at low levels of exposure, may contribute to the development or progression of the condition.
· Heavy Metals: Heavy metals like lead, known for their neurotoxic effects, have also been linked to PD when encountered in the environment.
· Solvents: Exposure to certain solvents such as trichloroethylene (TCE) and perchloroethylene (PERC), have been studied in relation to PD risk. Occupational exposure to these solvents has been associated with an increased likelihood of developing the condition.
· Mycotoxins 24; toxic compounds produced by certain moulds, have been investigated for their role in PD development. Some of the more extensively investigated mycotoxins include:
o Aflatoxins: Aflatoxins are produced primarily by Aspergillus species of moulds and are commonly found in nuts, grains, and legumes, as well as in milk and dairy products from animals consuming contaminated feed. Aflatoxins are potent carcinogens and have also been implicated in neurotoxic effects.
o Ochratoxin A: This mycotoxin is produced by Aspergillus and Penicillium and is commonly found in cereals, coffee, dried fruits, and wine. Ochratoxin A has been associated with potential neurotoxicity.
o Trichothecenes: Trichothecenes are produced by several moulds, including species of Fusarium and Stachybotrys. These mycotoxins can contaminate grains, especially in humid and warm conditions. They have been studied for their neurotoxic effects.
Phase 2 liver detoxification:
In addition to identifying and reducing exposure to environmental toxins, it is important to consider the individual’s capacity to bio transform toxins via the detoxification pathways. A reduction in phase 2 detoxification is particularly relevant in PD. Studies have shown that patients have reduced capacity to detoxify xenobiotics in particular. It is thought that reduced detoxification function can increase susceptibility to neurotoxins. Reduced liver detoxification is also associated with a higher level of free radicals and inflammatory cytokines.
Iron 25
Iron is involved in many fundamental biological processes in the brain including oxygen transportation, DNA synthesis, mitochondrial respiration, myelin synthesis and neurotransmitter synthesis and metabolism. Iron homeostasis is needed to maintain normal physiological brain function, whereas mis-regulation of iron homeostasis can cause neurotoxicity, and has been implicated in PD, where increased levels of iron in the substantia nigra have been detected. Oxidative damage to cells, resulting from the Fenton reaction of iron and the accumulation of iron in the substantia nigra, is associated with neuronal loss and Lewy body pathology. (The Fenton reaction = the oxidation of organic substrates by iron and hydrogen peroxide.) It is thought that the high iron content of the substantia nigra, required for dopamine synthesis, contributes to the vulnerability of the region and that iron supplementation may be associated with PD progression 26. Indeed, a 2022 large-scale study demonstrated that excessive supplemental iron intake was significantly associated with a higher risk of PD 27.
A nutritionally deficient diet 28
There is growing evidence to suggest that a nutritionally poor diet plays a significant role in the aetiology of PD. A diet lacking essential nutrients contributes to oxidative stress, inflammation, mitochondrial dysfunction, and impaired protein handling, all of which are implicated in the development and progression of PD.
One key factor is the consumption of processed and unhealthy foods high in sugars, refined vegetable oils and low in essential nutrients. Such a diet can lead to obesity and metabolic syndrome, both of which are associated with an increased risk of developing PD. Obesity is linked to chronic inflammation and insulin resistance which promotes neuroinflammation and oxidative stress in the brain, contributing to neuronal damage.
Antioxidant nutrients, such as vitamins C and E, beta-carotene, and polyphenols, play a crucial role in protecting the brain from oxidative stress. A diet lacking in these nutrients can reduce the body's ability to neutralise harmful free radicals, leading to increased oxidative damage to brain cells. Oxidative stress is believed to be a significant contributor to the loss of dopaminergic neurons in PD.
Deficiencies in certain vitamins and minerals will also influence the risk of developing PD. For example, inadequate intake of vitamin D has been associated with an increased risk of PD, as this nutrient plays a role in maintaining brain health and modulating the immune system.
Gut Health
Emerging research shows that gut health is linked to PD as both a cause and as a potential treatment option 29. A diet lacking in prebiotics and essential nutrients can negatively impact the gut microbiome, leading to dysbiosis (an imbalance in gut bacteria). Dysbiosis can trigger inflammation and the production of harmful metabolites that contribute to neuroinflammation and neurodegeneration in PD 30-32.
A holistic approach, including proper nutrition, regular exercise, and a healthy lifestyle, must be considered for the prevention and management/support of PD.
Other risk factors include:
· Gut parasites
· Viruses like HSV1, EBV
· Lyme’s disease
· Damage to basal ganglia by neurotoxins, such as glutamate
· Use of MPTP (designer heroin drug)
· Ageing
· Heavy metal toxicity
· Infection of central nervous system by mycobacterium
· High homocysteine, and reduced s-adenosylmethionine (SAMe)
· Nutrient deficiencies in the brain of zinc, COQ10, vitamin E, and selenium
Medical Treatment of Parkinson's Disease
PD remains without a cure, and current medical treatment aims to manage the symptoms effectively with a variety of medications. Medication plays a central role in this approach, with various drugs utilised, including:
Dopamine Replacement Drugs:
These medications aim to supplement dopamine levels in the brain, compensating for the reduced production by affected neurons.
Dopamine Agonists:
These drugs mimic the action of dopamine, stimulating dopamine receptors and alleviating the motor symptoms associated with PD.
Dopamine Metabolism Inhibitors:
Dopamine metabolism inhibitors are drugs that retard the degradation of dopamine, leading to an increased presence of this neurotransmitter in the brain.
Anticholinergic Drugs:
Anticholinergenic drugs inhibit the action of acetylcholine. Acetylcholine, a neurotransmitter, can worsen PD symptoms due to an imbalance between dopamine and acetylcholine in the brain. Increased acetylcholine levels can lead to motor disturbances and worsen tremors, rigidity, and bradykinesia, contributing to the characteristic motor impairments seen in PD.
Rasagiline & Selegiline:
Rasagiline is a medication used in PD management. It belongs to a class of drugs called MAO-B inhibitors, which help increase dopamine levels in the brain, improving motor symptoms and potentially slowing disease progression.
COMT:
Catechol-O-methyltransferase inhibitors are a class of medications used as adjunctive therapy in PD They work by inhibiting the COMT enzyme, which breaks down levodopa, a precursor to dopamine. By blocking COMT, these inhibitors prolong the effects of levodopa, reducing fluctuations in motor symptoms and enhancing its overall efficacy. COMT inhibitors can help manage motor complications and improve patients' quality of life when used in combination with levodopa therapy in advanced stages of PD.
Apomorphine:
A dopamine agonist called apomorphine can be injected under the skin (subcutaneously) either by a single injection, when required, or a continuous infusion using a small pump carried around on your belt, under your clothing or in a bag.
Co-careldopa:
For severe PD, a type of levodopa called co-careldopa may be pumped continuously into your gut through a tube inserted through your abdominal wall. There's an external pump attached to the end of the tube, which you carry around with you.
Given the diverse nature of symptoms among individuals, responses to medication can vary significantly. Finding the most suitable and effective treatment for each patient may require time and adjustments. Those in the early stages of PD usually respond well to treatment, but as the disease progresses, symptom management becomes more challenging, often necessitating increased dosages or the addition of alternative medications.
In addition to drug therapies, surgical procedures are sometimes employed to address specific symptoms such as tremors. These interventions aim to alleviate symptoms and improve the patient's quality of life. An example is Deep Brain Stimulation (DBS); a surgical treatment for advanced PD. Electrodes are implanted into specific brain regions, and electrical impulses are delivered to modulate abnormal neural activity, alleviating motor symptoms. DBS can reduce tremors, rigidity, and dyskinesias, offering significant improvement in quality of life for selected patients.
Looking towards the future, research is continuously exploring novel treatment options. Stem cell therapy and gene therapy are promising avenues currently in their early stages of development, offering potential advancements in the management and potential future cure for PD.
While there is still much to learn and achieve in the realm of PD treatment, the ongoing efforts to improve current therapies and explore innovative, holistic and person-centred approaches brings hope for a brighter future for those living with this challenging condition.
Mucuna pruriens: A natural L-DOPA approach
Mucuna pruriens, also known as velvet bean, is a tropical legume that has caught the attention of practitioners and patients for its therapeutic effects in PD 33-35. It has a long history of traditional use in Ayurvedic medicine for various neurological and musculoskeletal disorders. The interest in Mucuna pruriens stems from its high natural content of levodopa (L-DOPA), the precursor to dopamine. Levodopa crosses the blood-brain barrier and gets converted into dopamine in the brain, compensating for the dopamine deficit caused by the degeneration of dopaminergic neurons in Parkinson's disease.
Mucuna pruriens contains levodopa in a natural form, and several studies have suggested that it offers certain advantages over the synthetic levodopa used in conventional PD treatment. One potential benefit is that Mucuna pruriens might have additional neuroprotective compounds that could support and preserve dopaminergic neurons.
In addition, Mucuna appears to contain a form of natural carbidopa, a peripheral decarboxylase inhibitor, meaning it prevents the conversion of levodopa into dopamine outside the brain. This is important because, when levodopa is taken orally, a significant amount of it is converted to dopamine in the bloodstream before it reaches the brain. This can lead to side effects like nausea and can reduce the efficacy of the dose of L-DOPA.
Several research studies have compared the effects of Mucuna pruriens against medical levodopa-carbidopa therapy. Some of those studies reported that Mucuna pruriens improved motor symptoms effectively and, in fact, had a longer-lasting benefit compared to standard pharmaceutical levodopa-carbidopa preparations.
Mucuna pruriens has been shown to exhibit some powerful effects when compared to the synthetic forms of L-DOPA. According to neurologist and Mucuna expert Dr Rafael González Maldonado, its onset of action occurs at around 34 minutes, which is significantly quicker than the average time observed with synthetic forms. Additionally, the plasma elevation of the active components in Mucuna pruriens is 110% higher than that of synthetic forms, leading to potentially enhanced therapeutic effects. Moreover, the 'on' phase, characterised by symptom relief and improved motor function, lasts approximately 37 minutes longer when using Mucuna pruriens than compared to its synthetic counterparts. These findings highlight the potential benefits of Mucuna pruriens as a valuable alternative for individuals with PD 36-39.
Mucuna pruriens standardisation
A major concern with using Mucuna pruriens is the variable and unpredictable levodopa content in different preparations and batches, which makes dosing challenging. Recent innovations in HPLC standardisation of the levodopa content in Mucuna have helped to change this and ensure consistent dosing and a regulated response. As with any complementary therapy option, it is crucial for individuals with Parkinson's disease to work closely with their healthcare team to make informed treatment decisions that best suit their individual needs and medical condition.
Nutritional contraindications when taking L-DOPA
Protein and L-DOPA fight for absorption
Patients taking L-DOPA medication should be aware of how protein and amino acids can interfere with its effective absorption in the digestive tract. To ensure optimal effectiveness, it is recommended to consume protein at least 2 hours away from taking L-Dopa medication. This can prove difficult with regular dosaging schedules like those utilised with Mucuna pruriens, a plant-based source of levodopa.
Vitamin B6
Taking vitamin B6 in conjunction with L-DOPA should be avoided as B6 can convert L-DOPA into dopamine before it crosses the blood-brain barrier. Dopamine cannot cross into the brain like L-DOPA can. This leads to a reduced efficacy of the medication.
An individual approach to the support of Parkinson’s Disease:
Diet, lifestyle, nutrition and environmental factors as part of a support protocol
Due to the multifactorial nature of PD and its distinct manifestations in individuals, relying on a single approach for prevention and support is insufficient and lacking in clinical skill. Instead, a thoughtfully crafted combination of therapies is essential to achieve the best possible outcomes.
Overview:
When addressing prevention and support for PD, a comprehensive approach must consider several factors, such as:
· Dietary intervention with a view to removing foods that might have a negative impact on PD and focusing on adding foods that are nutritionally dense and supportive.
· Nutritional supplementation based on deficiencies discovered through blood and urine testing, or to ensure optimal levels of key supportive nutrients.
· Lifestyle changes to minimise stress and optimise relaxation.
· Speech and language input to maintain vocal strength.
· Regular, safe, and careful exercise.
· Holistic dentistry and addressing potential mercury toxicity. Dental amalgam, which contains elemental mercury, has raised concerns about potential links to neurological disorders like PD. Genetic factors, mercury metabolism, and other environmental exposures contribute to the complex relationship 40.
· Traditional Chinese Medicine (TCM) has shown promise in positively impacting PD. Acupuncture, a key component of TCM, aims to balance the body's energy flow. Research suggests that acupuncture might alleviate PD symptoms like tremors, stiffness, and balance issues, possibly by enhancing dopamine release and modulating neural circuits. While not a standalone cure, integrating acupuncture into Parkinson's care could offer complementary benefits, potentially improving patients' quality of life and reducing medication side effects 41.
· Autogenic training may help alleviate stress and anxiety, which can worsen symptoms and reduce overall well-being. Additionally, it can promote better sleep and reduce muscle tension, easing some motor symptoms.
· Osteopathy and/or chiropractic review and treatment, if needed. Both therapies focus on musculoskeletal alignment and nervous system function. Osteopathy emphasises whole-body balance to improve mobility and reduce pain, aiding PD patients with movement challenges. Chiropractic care aims to correct spinal misalignments that could impact nerve signalling.
· Temporomandibular joint (TMJ) review by a dentist or osteopath and remedial action, if needed.
· Sleep apnoea review and remedial action.
· Regulating of environmental influences to remove mould and other toxins.
· Establish a regular daily bowel motion by increasing fluid intake, maintaining a good gut microbiome, setting a regular time to sit on the toilet and the use of magnesium citrate or a similar gentle stimulant. Caricol has also proven to be an effective agent .
In addition, the four elements listed below can play a pivotal role in influencing the disease's risk and daily patient experience, making them vital considerations in any therapeutic strategy. The recommendations that have proved effective guidelines in a holistic clinical approach are:
1. Never hungry
2. Never stressed
3. Never tired
4. Exercise safely and regularly 42,43
Diet overview
In establishing a foundational dietary approach for the support and management of PD, it is crucial to adhere to the following guidelines that have a proven empirical effect in multiple clinical settings:
Certain foods have been shown to have a detrimental impact on PD symptoms. The list below is by no means complete and the PD patient should undertake an IgE and IgG food intolerance test to help guide them as to which foods to remove or reduce. The most common foods that have a negative impact on PD symptoms and should be avoided are:
Gluten 44
Cow's dairy (milk and cheese)
Butter and cream may be OK if well tolerated
If appropriate, avoid all forms of refined sugar. Avoid or radically reduce simple carbohydrates like white bread, rice, and pasta, and address any metabolic issues that impact on glucose uptake and use. 45,46 Consider testing for metabolic syndrome and type 2 diabetes.
If appropriate to the individual, avoid alcohol. Pay particular attention to high sugar, high yeast drinks like wine, beer, champagne and prosecco.
o Gin and vodka might be OK, but this is person specific. The general rule is if a certain type of alcohol degrades PD symptoms, then avoid it.
Carefully assess the impact of soya, corn, lentils, kidney beans, and peanuts on the individual’s PD symptoms. If a food or drink has a negative impact on movement, overall energy or cognition, then the suggestion is to refrain from consuming it for a few months.
If the protocol necessitates the removal of foods, make sure that alternatives are sought to replace the foods to be avoided. One of the dangers of dietary restrictions is that the PD individual can lose a significant amount of weight and become sarcopenic. Maintain a good daily calorie intake and make the dietary changes in a structured way over a comfortable period of time.
Additional dietary considerations:
In addition to the basics, certain essential elements should be included as a rule to enhance the supportive and healing effect of a nutritious diet:
Follow a plant-based organic diet, with 60% of your food intake comprising of largely non-starchy vegetables, either raw in salads or steamed. Dress liberally with extra virgin olive oil, avocado oil and apple cider vinegar. Season to taste.
Include low Glycaemic Index and load (GI/GL) fruits, with berries being an excellent option.
Focus on gluten free grains like quinoa, millet, buckwheat and tapioca for added nutritional variety.
Increase consumption of healthier fats with a focus on avocado, oily fish and fish oils, avocado oil, extra virgin olive oil, coconut oil, nuts and seeds.
Reduce consumption of inflammatory omega 6 fatty acids found mainly in vegetable oils (sunflower, rapeseed, margarine).
Regularly consume fermented foods such as Kimchi and Sauerkraut to promote a healthy gut microbiome.
Maintain a balanced, high NPU protein intake, with a daily portion of fish, free range eggs, tofu, tempeh, beef, beans/legumes or chicken.
Reduce exposure to refined vegetable or seed oils like sunflower and rapeseed.
Caloric restriction as an option 47,48
Caloric restriction is a potential strategy for addressing various conditions associated with ageing, and its applicability extends to PD prevention and as a support therapy. Studies indicate that caloric restriction can not only extend lifespan but also enhance the brain's resistance to insults involving metabolic compromise and excitotoxicity, both of which are relevant to PD pathogenesis.
Research suggests that calorie restriction promotes the activation of intrinsic pathways that lead to increased production of antioxidants. These antioxidants play a crucial role in combating oxidative stress, a process implicated in the degeneration of dopamine-producing neurons in PD. By bolstering antioxidant defences, caloric restriction may provide neuroprotective effects and help mitigate the progression of the disease.
Moreover, caloric restriction has been shown to modulate various cellular processes, such as autophagy, which involves the removal of damaged cellular components. This can aid in clearing out toxic protein aggregates, such as alpha-synuclein, whose accumulation is a mark of PD.
While the precise mechanisms by which caloric restriction confers its benefits in PD are still being explored, its potential as an adjunctive therapeutic approach is very promising. However, it's important to note that caloric restriction should be approached cautiously, as those with PD can lose weight rapidly and move into sarcopenia which is counter productive.
Intermittent fasting is perhaps an easier option 49,50
Based on an extended overnight fast, intermittent fasting might offer a more viable alternative to calorie-restriction. The benefits include:
Enhanced brain plasticity associated with increased levels of brain-derived neurotrophic factor (BDNF), a protein that supports brain health and plasticity. Improved brain plasticity may help the brain adapt to changes and potentially slow the progression of PD 51.
Regulation of insulin sensitivity as this form of fasting may improve insulin sensitivity and glucose metabolism, potentially reducing the risk of developing insulin resistance, a condition associated with an increased risk of neurodegenerative diseases like PD.
Nutritional intervention in PD
Introduction:
Several nutritional deficiencies have been associated with an increased risk of developing PD. Adequate vitamin D levels are essential for various neurological functions, and low vitamin D levels have been linked to a higher risk of PD. Vitamin B12 plays a crucial role in maintaining myelin and proper nerve function. Deficiencies in B12 have been associated with neurodegeneration and cognitive decline, which may contribute to the development of PD.
Folate is also important for neurological health. Low folate levels can lead to elevated homocysteine levels, which have been linked to an increased risk of PD. Deficiencies in antioxidants such as vitamin C, vitamin E, and selenium can impair the body's ability to combat oxidative stress, a key factor in PD pathogenesis. Antioxidants help protect neurons from damage caused by free radicals. Additionally, inadequate intake of omega-3 fatty acids and magnesium has been associated with an increased risk of PD. Omega-3 fatty acids play a role in brain health and inflammation regulation, while magnesium is involved in nerve function and protection against neurodegeneration.
Maintaining a balanced diet that includes adequate levels of these nutrients may help reduce the risk of developing PD.
Specific nutritional interventions in PD
Flavonoids 53,54
Flavonoids are a group of plant-derived polyphenolic compounds, which have garnered attention as potential supportive treatments for PD. Their diverse bioactive properties, such as antioxidant, anti-inflammatory, and neuroprotective effects, make them attractive candidates for managing neurodegenerative conditions like PD.
The antioxidant capacity of flavonoids plays a crucial role in combating oxidative stress, a prominent factor in the progressive degeneration of dopamine-producing neurons in PD. By neutralizing harmful free radicals and reducing cellular damage, flavonoids may help preserve neuronal function and slow down the disease progression. Moreover, flavonoids exhibit anti-inflammatory properties, which counteract neuroinflammation, a process we know is heavily implicated in the pathogenesis of PD. By modulating inflammatory responses within the brain, flavonoids may reduce neuronal damage and improve overall neuroprotection.
Certain flavonoids have demonstrated a capacity to cross the blood-brain barrier, allowing them to directly target brain tissues and exert their beneficial effects. These include compounds like quercetin, resveratrol, and curcumin, which have shown promise in preclinical studies and animal models of PD. In addition to their antioxidant and anti-inflammatory actions, flavonoids have been found to influence cellular signalling pathways involved in neuronal survival and synaptic plasticity. These effects could potentially enhance neuronal resilience and slow the decline in dopamine levels.
Instead of focusing on isolated substances, considering the combined effects of phytonutrients is essential. Specific flavonoids identified in research for their potential benefits in PD include:
· Catechins in green tea, which possess metal-chelating, antioxidant, and anti-inflammatory properties, benefiting neurons in the substantia nigra.
· Resveratrol and quercetin, which protect dopaminergic neurons by influencing the expression of genes related to apoptosis, the process of programmed cell death and the mechanism for removing dead and dying cells.
· Anthocyanins in mulberry fruit and blueberries, demonstrate neuroprotective effects in both in vitro and in vivo models of PD.
· Curcumin, with its ability to cross the blood-brain barrier, reducing reactive oxygen species and protecting against apoptosis. It shows promise for treating PD and other neurodegenerative diseases.
· Extracts from tangerine peel, cocoa, and red clover, found protective of dopaminergic neurons in animal studies.
· Flavonoids, especially polyphenols, exhibit membrane-stabilizing properties, contributing to the protection of mitochondrial and neural function.
Flavonoids seemingly hold great potential for supporting brain health in PD, and further investigation is needed to fully understand their mechanisms and therapeutic value. Adopting a diet rich in a variety of flavonoid-rich foods will provide a holistic approach to potential benefits for individuals living with PD.
Glutathione
Glutathione, a vital antioxidant synthesised in the body, plays a crucial role in neutralising harmful free radicals and protecting cells from oxidative stress. However, as we age, glutathione levels have been shown to decline, and patients with PD often exhibit reduced levels in the substantia nigra, a brain region affected by the condition. This deficit of glutathione may contribute to neuronal damage in PD.
To support glutathione levels, taking nutrients like N-acetylcysteine and alpha-lipoic acid will serve as precursors, facilitating its production. By providing these building blocks, these nutrients can offer the prescribed benefits in managing and ameliorating PD. Taking other antioxidants alongside the glutathione precursors can help conserve glutathione and enhance its protective effects. High doses of vitamin C in particular, have been shown to assist in preserving glutathione levels and bolster the body's antioxidant defences. Combining glutathione precursors with other antioxidants creates a synergistic effect, reinforcing the body's ability to combat oxidative stress and potentially mitigating the progression of PD disease.
Nattokinase 55
Nattokinase, an enzyme derived from fermented soybeans, has gained attention for its potential anti-inflammatory properties in PD. While research on its specific impact is limited, nattokinase's ability to modulate blood flow, reduce clotting, and target fibrinogen suggests potential anti-inflammatory effects.
NAC 56
N-acetylcysteine (NAC) has attracted attention in the context of PD due to its neuroprotective properties. As a precursor to glutathione, a critical antioxidant in the brain, NAC may help counteract oxidative stress and reduce neuronal damage in PD. Studies have shown that NAC supplementation can improve motor symptoms and delay the disease progression in animal models of PD. This could be significant in helping PD sufferers live more comfortably with the condition. Additionally, NAC's anti-inflammatory effects may contribute to its potential benefits in managing the condition.
COQ10 57
Coenzyme Q10 (CoQ10), a vital component of the mitochondrial electron transport chain and an antioxidant, has been investigated for its potential benefits in PD. Research suggests that CoQ10 may improve mitochondrial function and reduce oxidative stress, both of which are implicated in the pathogenesis of PD. A randomised, double-blind, placebo-controlled clinical trial found that CoQ10 supplementation was safe and showed a possible trend towards slowing the decline in functional status in early PD. However, more studies are needed to establish the definitive role of CoQ10 in PD management 58.
Vitamin D 59,60
Research on the relationship between vitamin D and PD has shown intriguing associations. Vitamin D, besides its role in bone health, possesses neuroprotective and anti-inflammatory properties that may be relevant in PD pathogenesis. Studies have indicated that low vitamin D levels are linked to an increased risk of developing PD and may contribute to disease progression. Vitamin D supplementation holds promise as a complementary approach in managing PD.
Vitamin E
Vitamin E, a fat-soluble antioxidant, has been a subject of interest in PD research. Oxidative stress and neuroinflammation are implicated in the disease's pathogenesis, and vitamin E's ability to neutralise free radicals and reduce inflammation may be beneficial. Studies have explored vitamin E supplementation as a potential neuroprotective intervention in PD. Recent findings suggest that dietary vitamin E and C intake might be inversely associated with the risk of PD 61.
Gingko Biloba 62
The use of Ginkgo biloba extract (GBE) in PD has been a subject of investigation due to its potential neuroprotective and antioxidant properties. GBE contains flavonoids and terpenoids that may scavenge free radicals and reduce oxidative stress, which are factors implicated in PD pathophysiology. Some preclinical studies and small clinical trials have suggested potential benefits of GBE in improving motor symptoms and cognitive function in Parkinson's patients. In a recent study, GBE treatment improved locomotor activity and inhibited the development of PD in the A53T α-synuclein transgenic mice, which may be partly responsible for decreased oxidative damage and maintain the normal dopamine homeostasis.
Curcumin 63
Curcumin, a natural polyphenolic compound found in turmeric, has garnered interest in PD research due to its potential neuroprotective and anti-inflammatory properties. Preclinical studies have demonstrated curcumin's ability to mitigate oxidative stress, reduce neuroinflammation, and inhibit the aggregation of alpha-synuclein, a protein associated with PD pathology. These findings have raised the possibility of curcumin as a therapeutic agent for PD. However, the limited bioavailability of curcumin in the brain remains a challenge. Clinical trials investigating curcumin's effects in PD are ongoing, with the hope of revealing its potential benefits in managing the disease. Further research is needed to validate curcumin's efficacy and establish appropriate dosages.
Ketone esters 64
The use of ketone esters in PD is an emerging area of research with promising potential. Ketone esters are compounds that raise blood ketone levels, providing an alternative fuel source for the brain and potentially improving neuronal energy metabolism. Preclinical studies in animal models of PD have shown encouraging results, suggesting ketone esters may enhance motor function, protect neurons, and reduce neuroinflammation. However, human clinical trials on the effects of ketone esters in PD are limited, and more extensive research is needed to determine their efficacy, safety, and optimal dosage for therapeutic use.
CBD 65
The use of CBD oil, a non-psychoactive compound derived from cannabis, in PD has been a topic of interest in recent research. Preclinical studies suggest that CBD may have neuroprotective effects, modulate neurotransmitter systems, and reduce neuroinflammation, which are relevant to PD pathogenesis. Some small clinical trials and anecdotal evidence have reported potential benefits of CBD in managing motor symptoms, sleep disturbances, and quality of life in PD patients. However, the evidence is still limited, and larger-scale, well-designed clinical trials are required to establish the safety, efficacy, and long-term effects of CBD oil as a therapeutic option for PD.
Taurine
Taurine, a sulphur-containing amino acid with various physiological roles, has shown potential implications in PD. Preclinical studies have suggested that taurine may demonstrate neuroprotective properties by reducing oxidative stress, modulating mitochondrial function, and inhibiting neuronal cell death pathways. These effects could be relevant in preserving dopamine-producing neurons in PD. Additionally, taurine has been investigated for its anti-inflammatory properties, which may contribute to its potential benefits in managing neuroinflammation associated with the disease. A recent study in mice showed that taurine exerted dopaminergic neuroprotection through inactivation of microglia-mediated neuroinflammation, providing a promising avenue and candidate for the potential therapy for patients suffering from PD66. However, research on taurine's effects in PD is still in its early stages, and further studies, including clinical trials, are needed to determine its therapeutic value and optimal dosing.
Vitamin B6 67
The use of vitamin B6 (pyridoxine) in PD is a subject of interest. While B6 is essential for various neurological functions, high doses may interfere with levodopa effectiveness, as B6 can convert levodopa into dopamine before crossing the blood-brain barrier. This conversion outside the brain hinders levodopa's intended therapeutic effect. PD patients should be cautious with B6 supplements and work closely with healthcare providers to determine appropriate dosages to avoid potential interactions with levodopa.
Low intake of vitamin B6 (pyridoxine) has been associated with an increased risk of PD. Vitamin B6 is crucial for various biochemical processes, including the metabolism of dopamine, a neurotransmitter involved in motor control. Dopamine is significantly affected in PD, and inadequate levels of B6 may impair dopamine synthesis and function. Moreover, B6 plays a role in protecting neurons from oxidative stress and inflammation, both of which are implicated in PD pathogenesis. Therefore, a deficiency in B6 may contribute to neurodegeneration and raise the risk of developing the condition. Maintaining an adequate intake of B6 through a balanced diet may help reduce the risk of this neurodegenerative disorder. However, further research is needed to establish the exact relationship between B6 intake and PD risk.
Vitamin B9 67
The role of folate, a water-soluble B-vitamin, in PD has been investigated due to its involvement in various biochemical processes relevant to neuronal health. Folate plays a crucial role in DNA and RNA synthesis, methylation reactions, and homocysteine metabolism. Low folate levels have been associated with elevated homocysteine levels, which have been implicated in neurodegeneration and increased risk of PD. Studies have shown that folate supplementation may help lower homocysteine levels and potentially offer neuroprotective effects. However, the evidence is inconclusive, and further research is needed to elucidate the precise relationship between folate and PD pathophysiology. [EW6]
Vitamin B12 67,68
Vitamin B12 (cobalamin) has been studied for its potential role in PD due to its involvement in various neurological processes. B12 is essential for maintaining myelin, the protective covering of nerve fibres, and proper nerve function. Low B12 levels have been associated with neurodegeneration and cognitive decline. Additionally, B12 is crucial for homocysteine metabolism, and elevated homocysteine levels have been linked to increased risk of PD. While some studies have suggested a potential association between B12 deficiency and PD, further research is needed to establish the exact role of B12 in the pathogenesis and management of Parkinson's.
Nutrients to consider in relation to Parkinson’s disease
Points to consider in the nutritional support of those diagnosed with Parkinson’s disease
Patient, Medical Practitioner and Nutritional Therapist communication to ensure that at all times the Medical Practitioner is aware and in agreement with any proposed dietary changes or supplement support.
2. Potential nutrient/drug interactions.
Nutrient | Examples of functions in relation to Parkinson’s disease |
Vitamin A | Vitamin A is a lipid antioxidant, therefore it is important for reducing oxidative stress within brain tissue which is predominantly comprised of fat |
Vitamin B | Major antioxidant and recycles glutathione, aids heavy metal detoxification and important for cell membrane integrity |
Vitamin B6 | Co-factor for production of dopamine (caution with levo-dopa) important for homocysteine methylation |
Folate | Methyl donor for homocysteine methylation |
B12 | Methyl donor for homocysteine methylation |
Vitamin D | Anti-inflammatory properties, has been shown to be insufficient in patients with Parkinson’s |
Vitamin E | Free radical scavenger and protects against lipid peroxidation |
Selenium | Protects cells from oxidative stress and can chelate heavy metals particularly mercury |
Zinc | Stimulates the antioxidant superoxide dismutase (SOD), protects cells from oxidative stress and contributes to normal brain function |
Alpha-lipoic acid | Antioxidant, supports mitochondrial function as a coenzyme for the production of energy |
CoQ10 | Cofactor in the electron transport chain therefore supports mitochondrial function and energy production and has antioxidant properties |
N-acetylcysteine | Antioxidant, stimulates glutathione synthesis and chelates heavy metals |
EPA/DHA | Anti-inflammatory properties (EPA precursor for anti-inflammatory prostaglandins), DHA contributes to normal brain function and development |
E | Anti-inflammatory as blocks COX-2 expression. Also has antioxidant, antiaging and neuroprotective effects and has potential to prevent Lewy body aggregation |
EPA/DHA | Antioxidant properties. Flavonoids also shown to have beneficial effects on neuro-inflammation and have metal chelating properties |
EPA/DHA | Enhances utilisation of oxygen and glucose, antioxidant and membrane stabilising effects |
Bibliography
References
1 Meireles J, Massano J. Cognitive impairment and dementia in Parkinson's disease: clinical features, diagnosis, and management. Front Neurol. 2012 May 25;3:88. doi: 10.3389/fneur.2012.00088. PMID: 22654785; PMCID: PMC3360424.
2 Yushi Kashihara et al. Effects of magnesium oxide on pharmacokinetics of L-dopa/carbidopa and assessment of pharmacodynamic changes by a model-based simulation. Eur J Clin Pharmacol. 2019 Mar;75(3):351-361. doi: 10.1007/s00228-018-2568-4. Epub 2018 Oct 31.
3 Bollu PC, Sahota P. Sleep and Parkinson Disease. Mo Med. 2017 Sep-Oct;114(5):381-386. PMID: 30228640; PMCID: PMC6140184.
4 Cerri S, Mus L, Blandini F. Parkinson's Disease in Women and Men: What's the Difference? J Parkinsons Dis. 2019;9(3):501-515. doi: 10.3233/JPD-191683. PMID: 31282427; PMCID: PMC6700650.
5 Dias V, Junn E, Mouradian MM. The role of oxidative stress in Parkinson's disease. J Parkinsons Dis. 2013;3(4):461-91. doi: 10.3233/JPD-130230. PMID: 24252804; PMCID: PMC4135313.
6 Jenner P. Oxidative stress in Parkinson's disease. Ann Neurol. 2003;53 Suppl 3:S26-36; discussion S36-8. doi: 10.1002/ana.10483. PMID: 12666096.
7 Chang, K.-H.; Chen, C.-M. The Role of Oxidative Stress in Parkinson’s Disease. Antioxidants 2020, 9, 597. https://doi.org/10.3390/antiox9070597
8 Dias, Vera, Junn, Eunsung, and Mouradian, M. Maral. ‘The Role of Oxidative Stress in Parkinson’s Disease’. 1 Jan. 2013 : 461 – 491.
9 N. Kolodkin, A., Sharma, R.P., Colangelo, A.M. et al. ROS networks: designs, aging, Parkinson’s disease and precision therapies. npj Syst Biol Appl 6, 34 (2020). https://doi.org/10.1038/s41540-020-00150-w
10 Smeyne M, Smeyne RJ. Glutathione metabolism and Parkinson's disease. Free Radic Biol Med. 2013 Sep;62:13-25. doi: 10.1016/j.freeradbiomed.2013.05.001. Epub 2013 May 8. PMID: 23665395; PMCID: PMC3736736.
11 Wang HL, Zhang J, Li YP, Dong L, Chen YZ. Potential use of glutathione as a treatment for Parkinson's disease. Exp Ther Med. 2021 Feb;21(2):125. doi: 10.3892/etm.2020.9557. Epub 2020 Dec 4. PMID: 33376507; PMCID: PMC7751460.
12 Asanuma, M.; Miyazaki, I. Glutathione and Related Molecules in Parkinsonism. Int. J. Mol. Sci. 2021, 22, 8689. https://doi.org/10.3390/ijms22168689
13 Zeevalk GD, Razmpour R, Bernard LP. Glutathione and Parkinson's disease: is this the elephant in the room? Biomed Pharmacother. 2008 Apr-May;62(4):236-49. doi: 10.1016/j.biopha.2008.01.017. Epub 2008 Mar 14. PMID: 18400456.
14 Wang Q, Liu Y, Zhou J. Neuroinflammation in Parkinson's disease and its potential as therapeutic target. Transl Neurodegener. 2015 Oct 12;4:19. doi: 10.1186/s40035-015-0042-0. PMID: 26464797; PMCID: PMC4603346.
15 Tansey, M.G., Wallings, R.L., Houser, M.C. et al. Inflammation and immune dysfunction in Parkinson disease. Nat Rev Immunol 22, 657–673 (2022). https://doi.org/10.1038/s41577-022-00684-6
16 Nalls MA, Pankratz N, Lill CM, Do CB, Hernandez DG, Saad M, DeStefano AL, Kara E, Bras J, Sharma M, Schulte C, Keller MF, Arepalli S, Letson C, Edsall C, Stefansson H, Liu X, Pliner H, Lee JH, Cheng R; International Parkinson's Disease Genomics Consortium (IPDGC); Parkinson's Study Group (PSG) Parkinson's Research: The Organized GENetics Initiative (PROGENI); 23andMe; GenePD; NeuroGenetics Research Consortium (NGRC); Hussman Institute of Human Genomics (HIHG); Ashkenazi Jewish Dataset Investigator; Cohorts for Health and Aging Research in Genetic Epidemiology (CHARGE); North American Brain Expression Consortium (NABEC); United Kingdom Brain Expression Consortium (UKBEC); Greek Parkinson's Disease Consortium; Alzheimer Genetic Analysis Group; Ikram MA, Ioannidis JP, Hadjigeorgiou GM, Bis JC, Martinez M, Perlmutter JS, Goate A, Marder K, Fiske B, Sutherland M, Xiromerisiou G, Myers RH, Clark LN, Stefansson K, Hardy JA, Heutink P, Chen H, Wood NW, Houlden H, Payami H, Brice A, Scott WK, Gasser T, Bertram L, Eriksson N, Foroud T, Singleton AB. Large-scale meta-analysis of genome-wide association data identifies six new risk loci for Parkinson's disease. Nat Genet. 2014 Sep;46(9):989-93. doi: 10.1038/ng.3043. Epub 2014 Jul 27. PMID: 25064009; PMCID: PMC4146673.
17 Day, J.O.; Mullin, S. The Genetics of Parkinson’s Disease and Implications for Clinical Practice. Genes 2021, 12, 1006. https://doi.org/10.3390/genes12071006
18 Moon HE, Paek SH. Mitochondrial Dysfunction in Parkinson's Disease. Exp Neurobiol. 2015 Jun;24(2):103-16. doi: 10.5607/en.2015.24.2.103. Epub 2015 Jun 8. PMID: 26113789; PMCID: PMC4479806.
19 Park JS, Davis RL, Sue CM. Mitochondrial Dysfunction in Parkinson's Disease: New Mechanistic Insights and Therapeutic Perspectives. Curr Neurol Neurosci Rep. 2018 Apr 3;18(5):21. doi: 10.1007/s11910-018-0829-3. PMID: 29616350; PMCID: PMC5882770.
20 Goldman SM. Environmental toxins and Parkinson's disease. Annu Rev Pharmacol Toxicol. 2014;54:141-64. doi: 10.1146/annurev-pharmtox-011613-135937. Epub 2013 Sep 16. PMID: 24050700.
21 Caudle WM, Guillot TS, Lazo CR, Miller GW. Industrial toxicants and Parkinson's disease. Neurotoxicology. 2012 Mar;33(2):178-88. doi: 10.1016/j.neuro.2012.01.010. Epub 2012 Jan 30. PMID: 22309908; PMCID: PMC3299826.
22 Najm Alsadat Madani, David O. Carpenter, Effects of glyphosate and glyphosate-based herbicides like Roundup™ on the mammalian nervous system: A review, Environmental Research, Volume 214, Part 4, 2022, 113933, ISSN 0013-9351, https://doi.org/10.1016/j.envres.2022.113933.
23 Eriguchi M, Iida K, Ikeda S, Osoegawa M, Nishioka K, Hattori N, Nagayama H, Hara H. Parkinsonism Relating to Intoxication with Glyphosate. Intern Med. 2019 Jul 1;58(13):1935-1938. doi: 10.2169/internalmedicine.2028-18. Epub 2019 Feb 25. PMID: 30799335; PMCID: PMC6663540.
24 Arce-López B, Alvarez-Erviti L, De Santis B, Izco M, López-Calvo S, Marzo-Sola ME, Debegnach F, Lizarraga E, López de Cerain A, González-Peñas E, Vettorazzi A. Biomonitoring of Mycotoxins in Plasma of Patients with Alzheimer's and Parkinson's Disease. Toxins (Basel). 2021 Jul 10;13(7):477. doi: 10.3390/toxins13070477. PMID: 34357949; PMCID: PMC8310068.
25 Ward RJ, Zucca FA, Duyn JH, Crichton RR, Zecca L. The role of iron in brain ageing and neurodegenerative disorders. Lancet Neurol. 2014 Oct;13(10):1045-60. doi: 10.1016/S1474-4422(14)70117-6. PMID: 25231526; PMCID: PMC5672917.
26 Mischley LK, Lau RC, Bennett RD. Role of Diet and Nutritional Supplements in Parkinson's Disease Progression. Oxid Med Cell Longev. 2017;2017:6405278. doi: 10.1155/2017/6405278. Epub 2017 Sep 10. PMID: 29081890; PMCID: PMC5610862.
27 Takeuchi H, Kawashima R. A Prospective Study on the Relationship between Iron Supplement Intake, Hemoglobin Concentration, and Risk of Parkinsonism. Nutrients. 2022 Nov 4;14(21):4671. doi: 10.3390/nu14214671. PMID: 36364932; PMCID: PMC9655462.
28 Seidl SE, Santiago JA, Bilyk H, Potashkin JA. The emerging role of nutrition in Parkinson's disease. Front Aging Neurosci. 2014 Mar 7;6:36. doi: 10.3389/fnagi.2014.00036. PMID: 24639650; PMCID: PMC3945400.
29 Zhu M, Liu X, Ye Y, Yan X, Cheng Y, Zhao L, Chen F, Ling Z. Gut Microbiota: A Novel Therapeutic Target for Parkinson's Disease. Front Immunol. 2022 Jun 24;13:937555. doi: 10.3389/fimmu.2022.937555. PMID: 35812394; PMCID: PMC9263276.
30 Romano, S., Savva, G.M., Bedarf, J.R. et al. Meta-analysis of the Parkinson’s disease gut microbiome suggests alterations linked to intestinal inflammation. npj Parkinsons Dis. 7, 27 (2021). https://doi.org/10.1038/s41531-021-00156-z
31 Li Z, Liang H, Hu Y, Lu L, Zheng C, Fan Y, Wu B, Zou T, Luo X, Zhang X, Zeng Y, Liu Z, Zhou Z, Yue Z, Ren Y, Li Z, Su Q, Xu P. Gut bacterial profiles in Parkinson's disease: A systematic review. CNS Neurosci Ther. 2023 Jan;29(1):140-157. doi: 10.1111/cns.13990. Epub 2022 Oct 25. PMID: 36284437; PMCID: PMC9804059.
32 Safa Salim, Fatima Ahmad, Ayesha Banu, Farhan Mohammad, Gut microbiome and Parkinson's disease: Perspective on pathogenesis and treatment, Journal of Advanced Research, Volume 50, 2023, Pages 83-105, ISSN 2090-1232.
33 Mucuna pruriens in Parkinson disease. A double-blind, randomized, controlled, crossover study. Roberto Cilia, Janeth Laguna, Erica Cassani, Emanuele Cereda, Nicolò G. Pozzi, Ioannis U. Isaias, Manuela Contin, Michela Barichella, Gianni Pezzoli. Neurology Aug 2017, 89 (5) 432-438; DOI: 10.1212/WNL.0000000000004175
34 Katzenschlager R, Evans A, Manson A, et al. Mucuna pruriens in Parkinson’s disease: a double blind clinical and pharmacological study. Journal of Neurology, Neurosurgery & Psychiatry 2004;75:1672-1677.
35 Maldonado RG (2018) Mucuna and Parkinson’s Disease: Treatment with Natural Levodopa. Parkinson’s Disease - Understanding Pathophysiology and Developing Therapeutic Strategies. InTech. DOI: 10.5772/intechopen.74062.
36 Ramya KB, Thaakur S. Herbs containing L- Dopa: An update. Anc Sci Life. 2007 Jul;27(1):50-5. PMID: 22557260; PMCID: PMC3330839.
37 Katzenschlager R, Evans A, Manson A, Patsalos PN, Ratnaraj N, Watt H, Timmermann L, Van der Giessen R, Lees AJ. Mucuna pruriens in Parkinson's disease: a double blind clinical and pharmacological study. J Neurol Neurosurg Psychiatry. 2004 Dec;75(12):1672-7. doi: 10.1136/jnnp.2003.028761. PMID: 15548480; PMCID: PMC1738871.
38 Lieu CA, Venkiteswaran K, Gilmour TP, Rao AN, Petticoffer AC, Gilbert EV, Deogaonkar M, Manyam BV, Subramanian T. The Antiparkinsonian and Antidyskinetic Mechanisms of Mucuna pruriens in the MPTP-Treated Nonhuman Primate. Evid Based Complement Alternat Med. 2012;2012:840247. doi: 10.1155/2012/840247. Epub 2012 Sep 10. PMID: 22997535; PMCID: PMC3445014.
39 Lieu CA, Kunselman AR, Manyam BV, Venkiteswaran K, Subramanian T. A water extract of Mucuna pruriens provides long-term amelioration of parkinsonism with reduced risk for dyskinesias. Parkinsonism Relat Disord. 2010 Aug;16(7):458-65. doi: 10.1016/j.parkreldis.2010.04.015. Epub 2010 May 31. PMID: 20570206; PMCID: PMC2909380.
40 Hsu YC, Chang CW, Lee HL, Chuang CC, Chiu HC, Li WY, Horng JT, Fu E. Association between History of Dental Amalgam Fillings and Risk of Parkinson's Disease: A Population-Based Retrospective Cohort Study in Taiwan. PLoS One. 2016 Dec 1;11(12):e0166552. doi: 10.1371/journal.pone.0166552. PMID: 27906991; PMCID: PMC5131949.
41 Zhang G, Xiong N, Zhang Z, Liu L, Huang J, Yang J, Wu J, Lin Z, Wang T. Effectiveness of traditional Chinese medicine as an adjunct therapy for Parkinson's disease: a systematic review and meta-analysis. PLoS One. 2015 Mar 10;10(3):e0118498. doi: 10.1371/journal.pone.0118498. PMID: 25756963; PMCID: PMC4355291.
42 Feng YS, Yang SD, Tan ZX, Wang MM, Xing Y, Dong F, Zhang F. The benefits and mechanisms of exercise training for Parkinson's disease. Life Sci. 2020 Mar 15;245:117345. doi: 10.1016/j.lfs.2020.117345. Epub 2020 Jan 22. PMID: 31981631.
43 Xu X, Fu Z, Le W. Exercise and Parkinson's disease. Int Rev Neurobiol. 2019;147:45-74. doi: 10.1016/bs.irn.2019.06.003. Epub 2019 Jun 20. PMID: 31607362.
44 Vinagre-Aragón A, Zis P, Grunewald RA, Hadjivassiliou M. Movement Disorders Related to Gluten Sensitivity: A Systematic Review. Nutrients. 2018 Aug 8;10(8):1034. doi: 10.3390/nu10081034. PMID: 30096784; PMCID: PMC6115931.
45 Souza APDS, Barros WMA, Silva JML, Silva MRM, Silva ABJ, Fernandes MSS, Santos MERAD, Silva MLD, Carmo TSD, Silva RKP, Silva KGD, Souza SL, Souza VON. Effect of Metabolic Syndrome on Parkinson's Disease: A Systematic Review. Clinics (Sao Paulo). 2021 Dec 13;76:e3379. doi: 10.6061/clinics/2021/e3379. PMID: 34909941; PMCID: PMC8634740.
46 Nam GE, Kim SM, Han K, Kim NH, Chung HS, Kim JW, Han B, Cho SJ, Yu JH, Park YG, Choi KM. Metabolic syndrome and risk of Parkinson disease: A nationwide cohort study. PLoS Med. 2018 Aug 21;15(8):e1002640. doi: 10.1371/journal.pmed.1002640. PMID: 30130376; PMCID: PMC6103502.
47 de Carvalho TS. Calorie restriction or dietary restriction: how far they can protect the brain against neurodegenerative diseases? Neural Regen Res. 2022 Aug;17(8):1640-1644. doi: 10.4103/1673-5374.332126. PMID: 35017409; PMCID: PMC8820686.
48 Luigi Fontana, Laura Ghezzi, Anne H. Cross, Laura Piccio; Effects of dietary restriction on neuroinflammation in neurodegenerative diseases. J Exp Med 1 February 2021; 218 (2): e20190086. doi: https://doi.org/10.1084/jem.20190086
49 Neth BJ, Bauer BA, Benarroch EE, Savica R. The Role of Intermittent Fasting in Parkinson's Disease. Front Neurol. 2021 Jun 1;12:682184. doi: 10.3389/fneur.2021.682184. PMID: 34140926; PMCID: PMC8203905.
50 Ojha U, Khanal S, Park PH, Hong JT, Choi DY. Intermittent fasting protects the nigral dopaminergic neurons from MPTP-mediated dopaminergic neuronal injury in mice. J Nutr Biochem. 2023 Feb;112:109212. doi: 10.1016/j.jnutbio.2022.109212. Epub 2022 Nov 10. PMID: 36370926.
51 Elesawy BH, Raafat BM, Muqbali AA, Abbas AM, Sakr HF. The Impact of Intermittent Fasting on Brain-Derived Neurotrophic Factor, Neurotrophin 3, and Rat Behavior in a Rat Model of Type 2 Diabetes Mellitus. Brain Sci. 2021 Feb 15;11(2):242. doi: 10.3390/brainsci11020242. PMID: 33671898; PMCID: PMC7918995.
53 Magalingam KB, Radhakrishnan AK, Haleagrahara N. Protective Mechanisms of Flavonoids in Parkinson's Disease. Oxid Med Cell Longev. 2015;2015:314560. doi: 10.1155/2015/314560. Epub 2015 Oct 20. PMID: 26576219; PMCID: PMC4630416.
54 Gao X, Cassidy A, Schwarzschild MA, Rimm EB, Ascherio A. Habitual intake of dietary flavonoids and risk of Parkinson disease. Neurology. 2012 Apr 10;78(15):1138-45. doi: 10.1212/WNL.0b013e31824f7fc4. Epub 2012 Apr 4. PMID: 22491871; PMCID: PMC3320056.
55 Xu et al. (2019). Nattokinase improves cognitive function and attenuates oxidative stress and neuroinflammation in Alzheimer's disease mouse model. Aging and Disease, 10(4), 949-963.
56 Monti DA, Zabrecky G, Kremens D, Liang TW, Wintering NA, Bazzan AJ, Zhong L, Bowens BK, Chervoneva I, Intenzo C, Newberg AB. N-Acetyl Cysteine Is Associated With Dopaminergic Improvement in Parkinson's Disease. Clin Pharmacol Ther. 2019 Oct;106(4):884-890. doi: 10.1002/cpt.1548. Epub 2019 Jul 17. PMID: 31206613.
57 Jiménez-Jiménez FJ, Alonso-Navarro H, García-Martín E, Agúndez JAG. Coenzyme Q10 and Parkinsonian Syndromes: A Systematic Review. J Pers Med. 2022 Jun 15;12(6):975. doi: 10.3390/jpm12060975. PMID: 35743757; PMCID: PMC9225264.
58 Shults CW, Oakes D, Kieburtz K, Beal MF, Haas R, Plumb S, Juncos JL, Nutt J, Shoulson I, Carter J, Kompoliti K, Perlmutter JS, Reich S, Stern M, Watts RL, Kurlan R, Molho E, Harrison M, Lew M; Parkinson Study Group. Effects of coenzyme Q10 in early Parkinson disease: evidence of slowing of the functional decline. Arch Neurol. 2002 Oct;59(10):1541-50. doi: 10.1001/archneur.59.10.1541. PMID: 12374491.
59 Barichella M, Garrì F, Caronni S, Bolliri C, Zocchi L, Macchione MC, Ferri V, Calandrella D, Pezzoli G. Vitamin D Status and Parkinson's Disease. Brain Sci. 2022 Jun 16;12(6):790. doi: 10.3390/brainsci12060790. PMID: 35741675; PMCID: PMC9221008.
60 Evatt ML, Delong MR, Khazai N, Rosen A, Triche S, Tangpricha V. Prevalence of vitamin d insufficiency in patients with Parkinson disease and Alzheimer disease. Arch Neurol. 2008 Oct;65(10):1348-52. doi: 10.1001/archneur.65.10.1348. PMID: 18852350; PMCID: PMC2746037.
61 Dietary Antioxidants and the Risk of Parkinson Disease. The Swedish National March Cohort. Essi Hantikainen, Ylva Trolle Lagerros, Weimin Ye, Mauro Serafini, Hans-Olov Adami, Rino Bellocco, Stephanie Bonn. Neurology Feb 2021, 96 (6) e895-e903; DOI:10.1212/WNL.0000000000011373
62 Kuang, S., Yang, L., Rao, Z., Zhong, Z., Li, J., Zhong, H., Tang, X. (2018). Effects of Ginkgo Biloba Extract on A53T α-Synuclein Transgenic Mouse Models of Parkinson’s Disease. Canadian Journal of Neurological Sciences, 45(2), 182-187. doi:10.1017/cjn.2017.268
63 Cortés H, Reyes-Hernández OD, Gonzalez-Torres M, Vizcaino-Dorado PA, Del Prado-Audelo ML, Alcalá-Alcalá S, Sharifi-Rad J, Figueroa-González G, González-Del Carmen M, Florán BN, Leyva-Gómez G. Curcumin for parkinson´s disease: potential therapeutic effects, molecular mechanisms, and nanoformulations to enhance its efficacy. Cell Mol Biol (Noisy-le-grand). 2021 Jan 31;67(1):101-105. doi: 10.14715/cmb/2021.67.1.15. PMID: 34817361.
64 Norwitz NG, Dearlove DJ, Lu M, Clarke K, Dawes H, Hu MT. A Ketone Ester Drink Enhances Endurance Exercise Performance in Parkinson's Disease. Front Neurosci. 2020 Sep 30;14:584130. doi: 10.3389/fnins.2020.584130. PMID: 33100965; PMCID: PMC7556340.
65 Vallée A, Vallée JN, Lecarpentier Y. Potential role of cannabidiol in Parkinson's disease by targeting the WNT/β-catenin pathway, oxidative stress and inflammation. Aging (Albany NY). 2021 Apr 13;13(7):10796-10813. doi: 10.18632/aging.202951. Epub 2021 Apr 13. PMID: 33848261; PMCID: PMC8064164.
66 Che, Y., Hou, L., Sun, F. et al. Taurine protects dopaminergic neurons in a mouse Parkinson’s disease model through inhibition of microglial M1 polarization. Cell Death Dis 9, 435 (2018). https://doi.org/10.1038/s41419-018-0468-2
67 Murakami K, Miyake Y, Sasaki S, Tanaka K, Fukushima W, Kiyohara C, Tsuboi Y, Yamada T, Oeda T, Miki T, Kawamura N, Sakae N, Fukuyama H, Hirota Y, Nagai M; Fukuoka Kinki Parkinson's Disease Study Group. Dietary intake of folate, vitamin B6, vitamin B12 and riboflavin and risk of Parkinson's disease: a case-control study in Japan. Br J Nutr. 2010 Sep;104(5):757-64. doi: 10.1017/S0007114510001005. Epub 2010 Mar 26. PMID: 20338075.
68 Shen L. Associations between B Vitamins and Parkinson's Disease. Nutrients. 2015 Aug 27;7(9):7197-208. doi: 10.3390/nu7095333. PMID: 26343714; PMCID: PMC4586528.
[RT1]Should we perhaps mention prebiotics here instead?
[RT2]Include some info about phospholipid form of glutathione
Write about difference between natural form tocopherols and tocotrienols compared to synthetic alpha tocopherol and the difference in function [EW3]
Optimised curcumin (longvida) is in a liposomal form which will cross the blood-brain barrier [EW4]
Outline difference between cyancobalamin and methlcobalamin active form - again we can help on this if needed [EW5]
Outline difference between folic acid and methylfolate - as folic acid will not lower homocysteine but methylfolate will - we can help on this if needed [EW6]